If you haven’t heard about time crystals, get ready to be wowed by physics – which is not a phrase I utter every day.
The main reason this discovery is so exciting is that they point to a whole new world of “non-equilibrium” phases that scientists have never even had the opportunity to study in the past. Most, if not all, matter (like metals and insulators) have been defined as being “in equilibrium” up to this point – which is a state where all the atoms in a material have the same amount of heat.
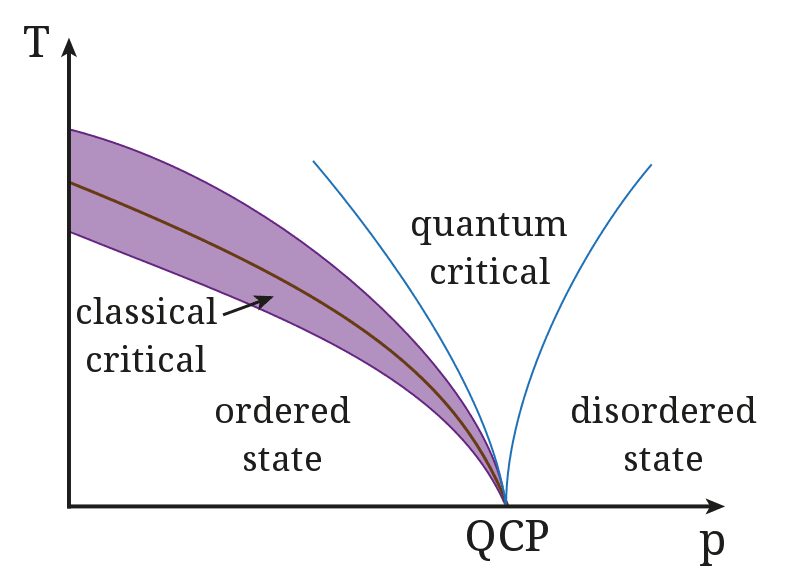
Image Credit: Public Domain
If time crystals exist in a “non-equilibrium” state of matter, they could revolutionize the way we store and transfer information via quantum systems, says physicist Norman Yao.
“It shows that the richness of the phases of matter is even broader than we thought.
One of the holy grails in physics is understanding what types of matter can exist in nature.
Non equilibrium phases represent a new avenue different from all the things we’ve studied in the past.”
Time crystals were first proposed by theoretical physicist Frank Wilczek back in 2012, hypothetical structures that appear to have movement even at their lowest energy state (known as a ground state).
Until then, movement in a ground state was theoretically impossible, because the material had no energy to expend. Wilczek, though, imagined an object that could continue to move by periodically switching the alignment of atoms inside the crystal over and over again, constantly moving in and out of the ground state at a rapid pace.
A zero energy object managing perpetual motion was one reason his hypothesis seemed unlikely, but there was another one, too – it hinted at a system that breaks one of the most fundamental understandings in physics.
Time-translation symmetry states that the laws of physics are the same everywhere and at all times. It’s the reason it would be impossible to flip a coin at one moment and have the odds of heads or tails at 50/50, but the next time, have the odds be 70/30, according to Daniel Oberhaus.
An object like a magnet, which is naturally asymmetrical with its north and south “poles,’ breaks this symmetry. It turns out, so do crystals.
Crystals have repeating structural patterns, but depending on where you observe one in space, it will look different – the laws of physics do not apply equally to all points in space.
This is the theory Wilczek used to suggest it might be possible to create an object that achieves an asymmetrical ground state not across space, but across time.
In short: could atoms prefer different states at different intervals in time?
Now, both American and Japanese researchers have shown this is possible, as long as you’re willing to allow for giving the crystals a nudge every once in a while to make them keep moving.
Norman Yao did his best to explain why this could be possible with no violation of physical laws.
“It’s like playing with a jump rope, and somehow our arm goes around twice, but the rope only goes around once.
It’s less weird than the first idea, but it’s still fricking weird.”
He commented on the two versions of time crystals that were created by two separate teams of researchers, calling both equally viable.
“Both systems are really cool. They’re kind of very different. I think they’re extremely complementary. I don’t think one is better than the other. They look at two different regimes of physics.
The fact that you’re seeing this similar phenomenology in very different systems is really amazing.”
Pretty trippy, right?
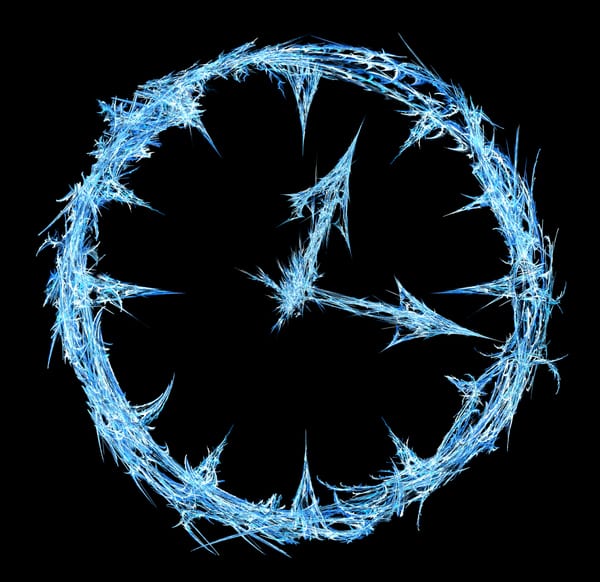
Image Credit: iStock
Fiona MacDonald, of Science Report, also broke down the breakthrough.
“The key to turning that set-up into a time crystal was to keep the ions out of equilibrium, and to do that the researchers alternately hit them with two lasers.
One laser created a magnetic field and the second laser partially flipped the spins of the atoms.”
The result was the spin-flipping pattern in the system only repeating half as fast as the laser pulses – like jiggling Jell-O and having it respond at different intervals, explained Yao.
The research has appeared in two separate papers (here’s the second one), so now the big question is, where do we find these things, and what do we do with them?
The most likely answer to the second question is quantum computing that could allow physicists to create stable quantum systems at higher temperatures than can currently be achieved – in fact, the time crystals might be the key to actually making it a reality.
As far as where we might find them, characteristics of time crystals have been found in a couple of unexpected places – a fertilizer compound and the crystal-growing kids you might have bought for your kids.
The compound is monoammonium phosphate, and its discovery is bringing up tough questions about how the crystals form in the first place.
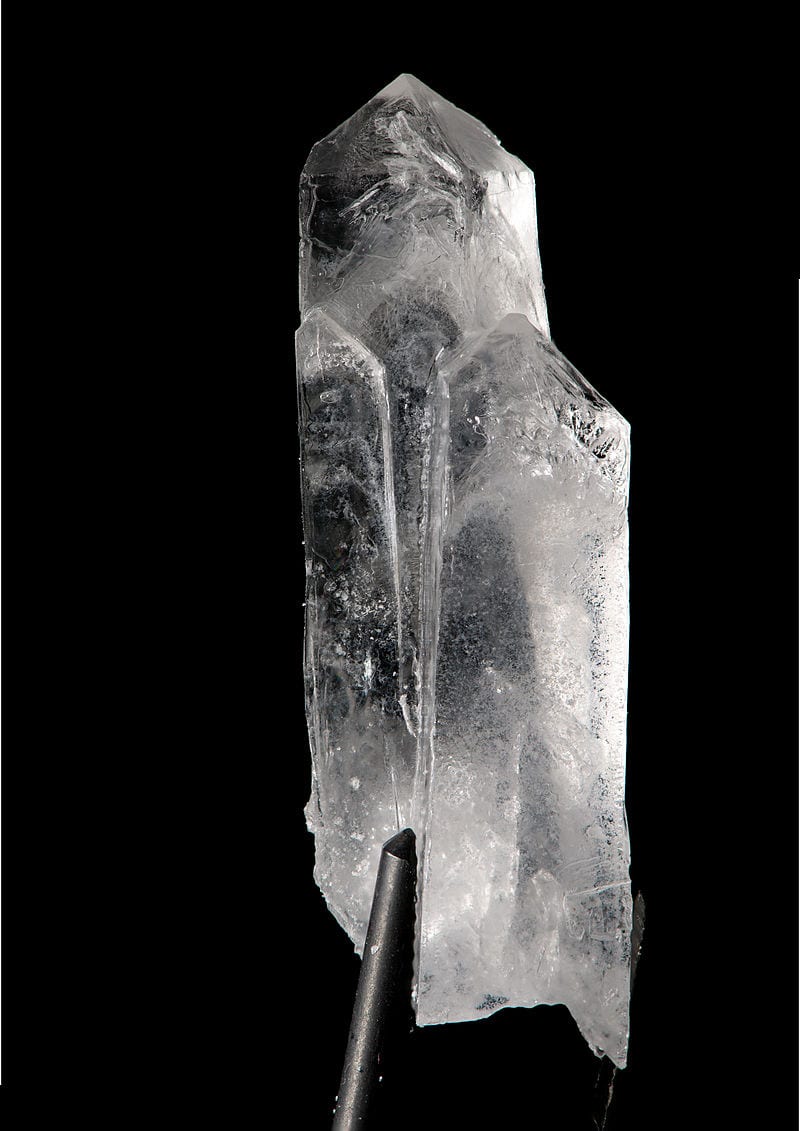
Image Credit: Damien Miller
To the naked eye, time crystals look like ordinary crystals, but as we’ve discussed, their atoms are actually oscillating instead of fixed. They spin one direction, then the other when exposed to an electromagnetic pulse.
Those oscillations are locked into a particular – and regular – frequency.
These new versions of time crystals are different from each of the others that was previously used to publish research on the subject, explained physicist Sean Barrett.
“We decided to try searching for the DTC signature ourselves.
My student Jared Rovny had grown monoammonium phosphate crystals for a completely different experiment, so we happened to have one in our lab.”
These crystals are easy to grow, and kits are literally everywhere in the world – which is one of the reasons finding time crystal signatures in such an ordered environment was odd.
“Our crystal measurements looked quite striking right off the bat.
Our work suggests that the signature of a DTC could be found, in principle, by looking in a children’s crystal growing kit.”
In addition to quantum computing, scientists are hoping for plenty of other practical applications like improving atomic clock technology, gyroscopes, and systems like GPS that rely on extremely accurate timekeeping.
The fact that they may be easier to find and manipulate than previously thought would certainly make all of that research and development easier, but Barrett warns there’s still plenty of work to do.
“It’s too early to tell what the resolution will be for the current theory of discrete time crystals, but people will be working on this question for at least the next few years.”
Here’s to science, friends.
I love that we truly never know what they’re going to stumble across next.